Intravascular Coils
The majority of all deaths in the United States result from cardiovascular disease. The rupture of an atherosclerotic plaque is often suspected to be the event precipitating a heart attack or stroke. Magnetic resonance imaging (MRI) and MR spectroscopy (MRS) are playing an increasing role in the identification and characterization of potentially vulnerable plaque, but often exhibit marginal spatial resolution and signal to noise ratio (SNR) in this role. Conventional MR scanning employs radiofrequency (RF) coils (the transducers for MR signals) positioned to cover the scanning region of interest, but always external to the body. Intravascular MR coils, which can be inserted into blood vessels and placed in close proximity to vessel walls, offer the promise of greatly improved SNR, but have not attained the desired performance characteristics to be clinically useful due to a variety of problems: (1) Optimum sensitivity is obtained when the sensitive volume of the coil matches the scanning volume of interest. External coils are too far from and much larger than plaques; (2) Previous intravascular coils have maximum sensitivity in the lumen of the artery and minimum sensitivity at the arterial wall where the plaques are, thereby swamping the plaque signals; (3) Because of their small size and inaccessibility, intravascular coils, once positioned, cannot be tuned with variable mechanical capacitors; (4) The long, small diameter coaxial cable leads to significant signal loss. We are adopting a variety of approaches to overcome these problems, including the design of novel intravascular coils with sensitive volumes tailored to the cylindrical arterial wall geometry, as well as incorporating electrically variable tuning and matching capacitors directly at the iv coil which may be remotely adjusted with DC voltages, and finally incorporating miniature preamplifiers inside the catheter in close proximity to the coil.
1. Cylindrical Meanderline RF Coil:
Intravascular RF coils are being investigated by several research groups to improve the filling factor and signal to noise ratio for NMR imaging and spectroscopy of atherosclerotic plaque. These coils are introduced percutaneously into blood vessels via catheters in much the same way as are surgical devices used for opening clogged arteries. In order to obtain the best filling factor in such applications we are investigating the use of novel RF coil structures with B1 field profiles tailored to the geometry of the arterial wall. Cylindrical meanderline coils provide a sensitive detection volume that is restricted to a cylindrical shell, thereby maximizing the filling factor for the vessel wall, and plaques in particular, while reducing the blood signal.
 |
Figure 1: Planar (left) and cylindrical (right) meanderline coil designs. The thickness of the sensitive volume is determined by the spacing between the conductors. |
Wrapping a meanderline into a cylinder (Figure 1) takes advantage of the structure’s tight B1 shaping properties, which should minimize blood flow image artifacts and increase the dynamic range available from plaque signals. The antiparallel alignment of adjacent conductors of the meanderline coil insures that B1 falls off rapidly with distance from the surface of the coil. This behavior is demonstrated in Finite Difference Time Domain (FDTD) simulations of the electromagnetic field (Figure 2) of a 3 mm o.d. cylindrical meanderline coil, where the region of the most intense RF field is restricted to a thin cylindrical shell. However, a more distorted, crescent shaped, field symmetry is observed with increased loading of the coil with saline (σ = 1.4, ε = 70).
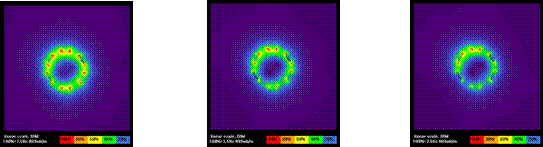 |
Figure 2: FDTD simulations of a 3 mm meanderline coil in (a) free space, (b) immersed in saline with the coil interior empty, and (c) immersed in saline with the coil interior full. |
Shown in Figure 3 are prototype 3 mm and 2 mm o.d. meanderline coils (6 conductors, 20 mm long, 6 mm wide, 1.6 mm spacing ) used to acquire experimental images. The coils are connected to a tuning and matching pi-circuit via small diameter 50 ohm coaxial cable (1.8 mm o.d.) of length ~λ/2. Experimental images acquired with the 3 mm meanderline coil, shown in Figure 4, are in good qualitative agreement with the FDTD simulations.
 |
 |
Figure 3: Close-up views of 3 mm (a) and a 2 mm (b) o.d. cylindrical meanderline coils. The coils are produced by etching copper clad Kapton polyimide flexible printed circuit substrate. The pictured coils contain six conductors and have inductances on the order of 50 nanohenries. |
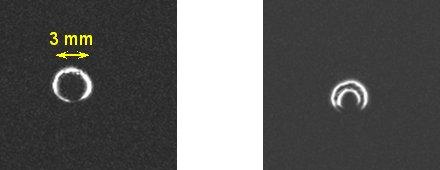 |
Figure 4: Image acquired with a 3 mm diameter meanderline coil immersed in 1.0 mM MnSO4 doped water with the coil interior either empty (left) or filled (right). The field of view is 20 mm and the in -plane resolution is 0.16 mm. |
The distortion of the field symmetry observed both in the simulations and experimentally can be minimized by incorporating tuning capacitance into the meanderline coil as demonstrated in both the FDTD simulations of the magnitude of the magnetic field and the experimental data shown in Figure 5.
 |
 |
 |
 |
Figure 5: FDTD simulations of a 3 mm od cylindrical meanderline coil without (top, left) and with six 60 pF tuning capacitor distributed in into the coil. The simulations are in very good agreement with the experimental images obtained without any local tuning capacitor (bottom, left) and with a 10 pF tuning capacitor (bottom, right). |
Finally, images of an endarterectomy specimen (see Figure 6) were acquired at 4.7 Tesla with a 127 mm diameter bird-cage volume coil and a 3 mm meanderline surface used in receive mode. The SNR is clearly superior for the image acquired with the receive-only cyclindrical meanderline coil over that obtained with the transmit-receive volume coil.
 |
Figure 6: Endarterectomy images acquired at 4.7 T with (left) a 127 mm bird-cage coil and (right) a 3 mm meanderline coil. The FOV was 25 mm with a 1 mm slice thickness and a 0.1 mm in plane resolution. The red circle represents the position of the meanderline coil in the endarterectomy specimen. |
These preliminary studies demonstrate that a cylindrical meanderline coil provides a cylindrical shell sensitive volume that should be well suited for imaging blood vessel walls and atherosclerotic plaque. The cylindrical meanderline coil design provides several potential advantages over other more commonly employed catheter coil designs: (a) the meanderline coil sensitive volume is limited to a cylindrical shell, allowing for the selective imaging of arterial walls with minimal or no signal from blood, thereby reducing dynamic range problems due to the intense blood signal; (b) the more restricted volume of sensitivity of the meanderline coil reduces the noise picked up by the coil due to the highly conductive blood, thereby improving the SNR; (c) because of its readily conformable and expandable geometry, the cylindrical meanderline coil could be made from shape-persistent materials or expanded with an annular balloon allowing it to be deployed similarly to a stent, thereby matching its diameter with that of the blood vessel wall and minimizing imaging artifacts due to coil motion during pulsatile blood flow by stabilizing the coil against the vessel wall; (d) the center of the coil is open and allows for unimpeded blood flow during image acquisition; (e) since the coil B1 field is always perpendicular to the long axis of the coil cylinder there will always be some component of the coil B1 field perpendicular to the applied field B0 for any orientation of the coil and hence a signal will always be observed. In contrast, signal dropout is observed with other coil designs where, due to blood vessel tortuosity, some coil orientations result in the coil’s B1 field (or axis of sensitivity) being oriented parallel to the applied static magnetic field.
2. Local IV Coil Tuning, Matching, and Signal Preamplification:
In collaboration with Draper Laboratory we have initiated a project to design miniaturized coil tuning, matching, and preamplification circuitry directly at the iv coil to maximize the SNR. The functionality includes an ultra low noise transistor amplifier stage, a PIN diode to detune the RF coil and protect the transistor during RF pulses, and a pair of varactor diodes (electrically controllable capacitors) which can be used to tune the coil remotely (see Figure 7). The active components (marked by the white rectangle in Figure 7) occupy a thin strip about 2 mm in width, and with some modification of this circuit could be placed within a 7F catheter.
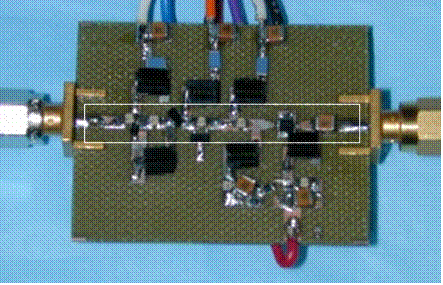 |
Figure 7: Prototype tuning, matching, and preamplification circuit |
|